Optimizing horizontal well placement is not limited to identifying the most-favorable reservoir, but also involves identifying the ideal target window within that reservoir. Gathering drill-bit geomechanics data provides a lower-cost and lower-risk method to acquire mechanical rock properties in long horizontal wellbores. By integrating data sets with mechanical rock properties recorded while drilling, operators can have significantly higher confidence in choosing a target landing zone and improving completions. The complete paper presents two detailed case studies from the Permian Basin.
Introduction
In this paper, the authors combine the characterization of petrophysical and geomechanical properties into what they call a petromechanical work flow.
×
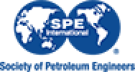
Continue Reading with SPE Membership
SPE Members: Please sign in at the top of the page for access to this member-exclusive content. If you are not a member and you find JPT content valuable, we encourage you to become a part of the SPE member community to gain full access.