This paper describes the use of a downhole temperature-sensor array during a commingled drillstem test (DST) to determine the density of produced fluids accurately. In a typical DST that uses only downhole pressure gauges, any fluid contacts between the pressure gauges would be missed and the produced-fluid density calculated would be erroneous. The complete paper demonstrates the importance of taking fluid properties into account when determining the zonal flow-rate contributions using the mass-enthalpy method.
Introduction
Downhole temperature-sensor-array data provide accurate fluid contact depths during buildup periods of the DST that typically cannot be observed in pressure gradients. Determination of these fluid contacts permits the calculation of individual produced-fluid densities.
In a case study described in the complete paper, the deepest perforated zone produced a fluid with a higher density than that seen in shallower perforated intervals.
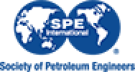