A demonstration project of carbon capture, utilization, and storage (CCUS) through enhanced oil recovery (EOR) was conducted in Saudi Arabia. The main objectives of the project are quantifying the amount of carbon dioxide (CO2) sequestered in the reservoir; addressing the risks and uncertainties involved, including remaining oil saturation and the migration of CO2 within the reservoir; identifying operational concerns; and estimating the incremental oil recovery (beyond waterflooding). This paper covers the details of the overall facilities design.
Introduction
CO2 is a great injectant for EOR—for environmental, technical, and economic reasons. It is an excellent solvent, especially for light crudes, and can considerably enhance overall oil recovery. CO2 swells the oil and reduces oil viscosity significantly.
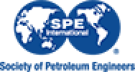